1. Introduction
The use of Life Cycle Assessment (LCA) to understand environmental impacts can significantly facilitate decision-making when selecting a design option, provided that the chosen design minimizes environmental impact throughout its lifetime. The objective is to select a building subsystem option by assessing life-cycle impacts and utilizing Multi-Criteria Decision-Making method AHP. As a subsystem a slab is chosen for a community multipurpose building. (The references are given in the Full Report at the end of the page.)
2. Goal and Scope
In this analysis, the scope extends from cradle to grave, encompassing the marked categories presented in the figure below. The functional unit for all elements, except for reinforcement, is m³, whereas for reinforcement, it is kg. The objective is to conduct an ecological footprint analysis, considering energy, CO2, PO4, and SO2 as criteria and the design options as the alternatives.
3. Design Options
Three possible slab design options are shown in Table 1. Material and dimensions are provided in Table 2.
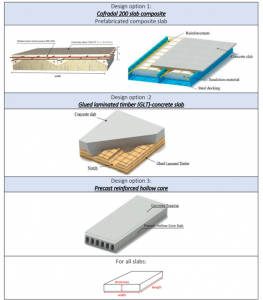
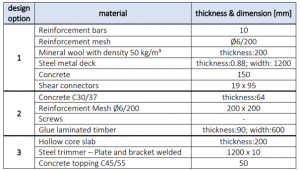
The table above contains the dimensions of the design options elements. For simplification, the analysis will not consider connectors such as screws, shear studs, and steel trimmers. All slabs have a span of 7 m and support a live load of 2.5 kN/m² over a 50-year period.
4. Life Cycle Inventory
The environmental indicators based on the slab materials were collected using the sources listed in the table below. To simplify the R implementation and address vector creation issues caused by diverse scopes of composite elements, they were combined into a single entity. It’s essential to recognize that each element has unique volume, environmental values, and functional units.
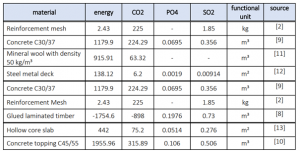
Two critical steps in computing the final values for the LCI will be briefly outlined. Concerning the hollow core slab, an additional assumption was made, and an equivalent thickness of the slab was calculated, encompassing only the concrete volume by deducting the hollow cores. The material for the slab itself and the concrete topping above it were selected to be identical.
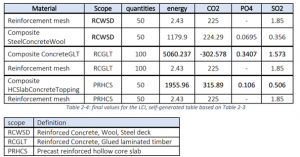
4. Life Cycle Timeline
Every design option requires material dependent interventions with specific frequency which as mentioned are going to be encountered in the LCA later on. The following table contains basic interventions for given event examples.
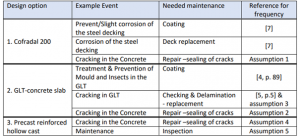
The frequencies of needed interventions, were set according to the references in the table and the described assumptions in Table 6.
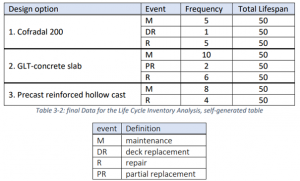
6. Life Cycle Analysis
The results of the Life Cycle Inventory Analysis were computed using R, which facilitated the determination of material quantities for each slab and the total material needed for every design option. Subsequently, the environmental indicators were applied to calculate the corresponding environmental impacts for each option. The findings are presented in the following barplots.
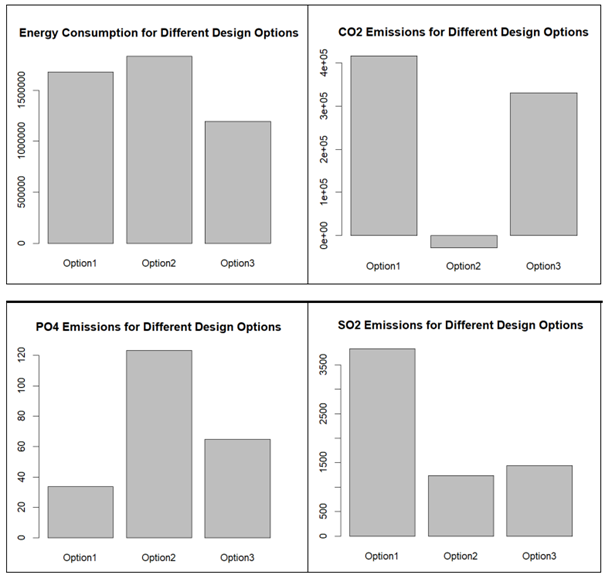
The environmental indicator that exhibits the most variation among the three design options is carbon emissions. Option 2 has the lowest, even a negative value, possibly influenced by the bio-chemical behaviour of wood over its lifetime and the very good recycling properties. Simultaneously, Option 2 shows the highest PO4 emissions. Energy consumption is comparable across all three options. The most even distribution of emissions is observed in design Option 3. Based on these plots alone, it becomes evident that achieving the goal of ranking the slabs based on ecological emissions depends on the weighting of the categories.
7. MCDM – Analytic Hierarchy Process
The AHP is employed for ranking slab options by assigning relative weights to criteria and alternatives, converting subjective assessments into ratio scales. The pairwise comparison of alternatives is facilitated using barplots in Table 8, with Saaty’s scale used for weighting.
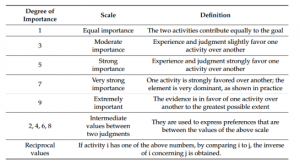
Since CO2 is the main key indicator according to the organization for economic and co-operation (OECD), it will be assigned the highest weight, followed by SO2 and energy based on their ranking. Since PO4 is not considered a key indicator, it will carry the least weight.
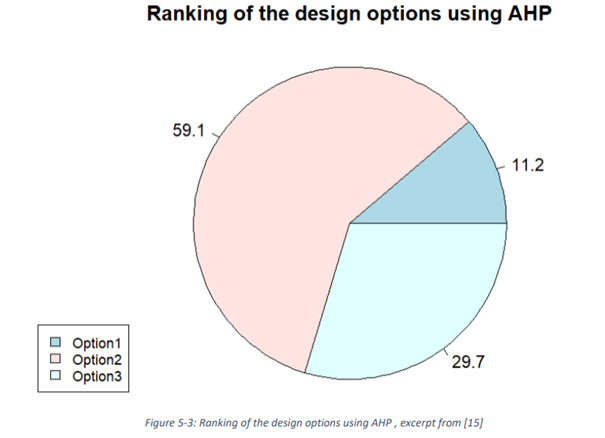
Regarding the results, it seems that Option 2 – the GLT concrete slab is the most favourable choice. This is likely because CO2 was highly prioritized in the pairwise comparison of the criteria. The precast hollow core slab appears to be a better option than the Cofradal slab. While the results are satisfactory based on the current ranking and scope, they can change when specific or varied goals are considered.
In conclusion, using the information provided in the assignment and considering the set goal and scope, the “best” option depends on the weighted criteria. This underscores the multi-criteria nature of the decision problem. At this point, applying sensitivity analyses would be very useful.
Downloadable Content
Full Report: Tala Nada Fatima Ramadan