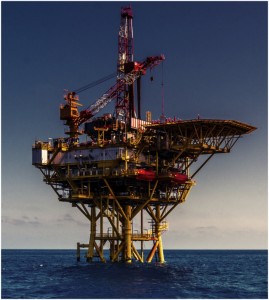
One of the structures in our integrated model is an off-shore structure with a jacket body. The system has three components, the jacket which is made of structural steel, the foundation that is made of reinforced concrete, and the pile foundations. Finally, a deck that is a combination of structural steel, and steel grating. Just like the supporting structures of the system, the deck could be made out of different components but the second option of steel girder beams, and steel grating was selected to keep the emission at the minimum in light of previous research.
Integrated Systems’ Affect on the Off-shore Structure
The main cause of failure and damage in offshore wind turbine support structures is fatigue and corrosion. Constant exposure to seawater accelerates material degradation, leading to localized corrosion, particularly at weld joints and splash zones (Ersdal, 2005; Jin and Mukherjee, 2014). Fatigue damage results from the continuous dynamic loads imposed by wind and wave interactions, which induce cyclic stresses on structural components. Unlike buildings on land, offshore structures are subjected to higher-frequency loading cycles, making their fatigue risk significantly greater. The expected design life of offshore wind turbine foundations, with current advancements in protective coatings and material improvements, is estimated to be around 80 years (Ersdal, 2005; Davies, 2018).
Cost Saving Due to the Integration
Fatigue in offshore structures from cyclic wave loading accelerates crack propagation, reducing structural integrity and service life (Jin and Mukherjee, 2014). Repair costs are substantial, with offshore coating repairs reaching €1000/m², up to 50 times the initial application cost (Price and Figueira, 2017). Fatigue-induced failures lead to expensive retrofits and downtime, significantly increasing operational costs.
Figure-2 and Figure-3 Example of an Offshore Structure Deck with Steel Grating
For the offshore structures, the core maintenance items are fatigue and corrosion. (Ersdal,2005). This effects the deck structure regardless of not being directly in the ocean water. The wind, wave impacts, and getting wet with ocean water and rain constantly increase fatigue, and corrosion damage, and the probability of failure. For this project we are considering the affects on the deck. Previously the best option for emissions and operational use, and ease of maintenance the steel grating with the steel girders were selected (Option-2).
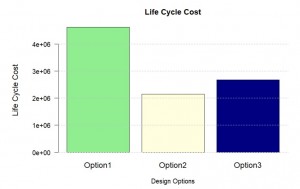
The bar chart compares the Life Cycle Cost (LCC) of three structural design options. Option 1 (Cast-in-Place Reinforced Concrete Slab + S355 Steel Girders) has the highest LCC, exceeding 4 million, making it the most expensive. Option 2 (Steel Girders + Steel Grating) has the lowest LCC, around 1.5 million, indicating it is the most cost-effective. Option 3 (Pre-cast Concrete Girders + Pre-cast Concrete Slab) falls in between, with a moderate LCC of around 2–3 million. This suggests that while Option 1 is the most costly, Option 2 is the most economical, and Option 3 provides a balance between cost and durability.
Reducing The Wave Horizontal Loading and Fatigue
Models illustrate that the estimated reduced wave load reduces the probability of failure due to the wave slightly. Also, making a slight positive impact on the aging of the structure, and need for maintenance to maintain the structural integrity.
According to Nguyen et al., the average wave energy reduction for different types of breakwaters is approximately 70%. Consequently, the model predicts a reduced impact on the wave horizontal loading related to aging, therefore probability of failure and the maintenance needed will also be reduced as well.
Additionally, the impact of a 100-year extreme wave event on offshore structures is estimated to decrease by 50%. The comparison below illustrates the differences between previous models and the new integrated model incorporating these adjustments. Comparing the probability of failure throughout the life-cycle.
Considering the breakwater’s support to the structure, the probability of failure and the need of maintenance due to the fatigue will reduce. These are evaluated below in R-studio with the updated probability of failures of Assignment 1.
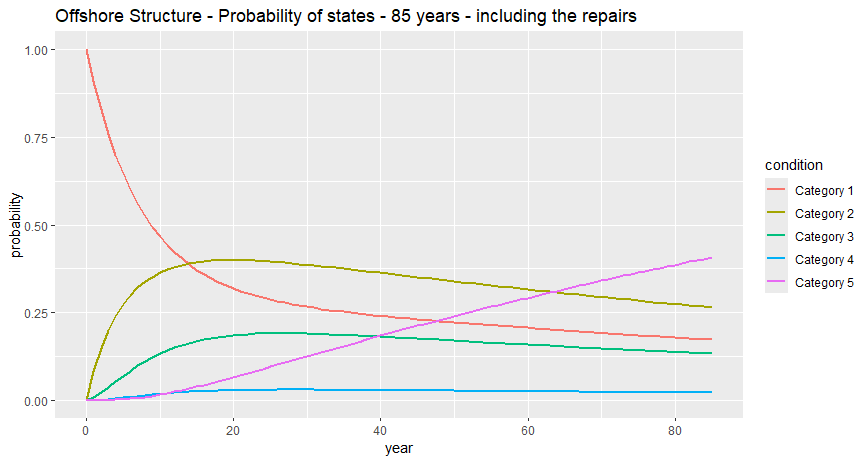
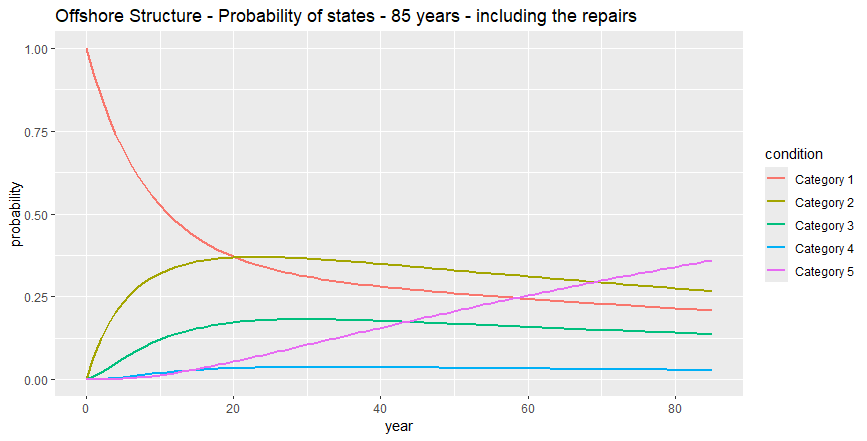
These graphs compare the degradation of an offshore structure over 85 years, with and without breakwater protection. Without a breakwater, the probability of the structure being in excellent condition (Category 1) sharply declines from 1.0 to around 0.4 by year 20, indicating rapid early deterioration due to harsh marine conditions. Subsequently, Category 2 becomes dominant, while Categories 3-5 gradually increase, reflecting progressive damage states. With breakwater protection, the initial probability of Category 1 remains near 100% and decreases much more slowly. Although the structure still degrades over time, the probabilities of poorer states (4 and 5) remain low even after 80 years, demonstrating the breakwater’s effectiveness in prolonging lifespan and maintaining integrity. This highlights the significant benefit of breakwater protection in mitigating structural deterioration.
References
• Zhang, Xiliang, et al. “A Review of Renewable Energy Policy and Market Development in China: A Need to Realign National Targets.” Renewable and Sustainable Energy Reviews, vol. 116, 2019, 109372. ScienceDirect, https://www.sciencedirect.com/science/article/pii/S0141118718301809. Accessed 4 Feb. 2025.
• Ersdal, Gerhard. Assessment of Existing Offshore Structures for Life Extension. Doctoral Thesis, University of Stavanger, Faculty of Science and Technology, 2005. ResearchGate, www.researchgate.net/publication/242462159.
• Ersdal, Gerhard. “On the Safety of Fixed Offshore Structures, Failure Paths and Barriers.” Proceedings of OMAE 2002, 21st International Conference on Offshore Mechanics and Arctic Engineering, 23-28 Jun. 2002, ASME. DOI: 10.1115/OMAE2002-28609.
• Sahasakkul, Watsamon, Hieu Nguyen, and Ali Sari. “Fatigue Reliability Assessment for Life Extension of Offshore Structures.” Offshore Technology Conference, May 2016, DOI: 10.4043/27280-MS.
• Davies, Louise. “Using an Offshore Platform Beyond Its Expected Lifespan.” Engineer Live, 1 Aug. 2018, www.engineerlive.com/content/using-offshore-platform-beyond-its-expected-lifespan. – Lifetime of offshore structures
•Jin, Yongliang, and Amlan Mukherjee. “Markov Chain Applications in Modelling Facility Condition Deterioration.” International Journal of Critical Infrastructures, vol. 10, no. 2, 2014, pp. 93–112. Inderscience Enterprises Ltd., doi:10.1504/IJCIS.2014.062965.
•Price, Seth J., and Rita Bacelar Figueira. “Corrosion Protection Systems and Fatigue Corrosion in Offshore Wind Structures: Current Status and Future Perspectives.” Journal of Marine Science and Engineering, vol. 5, no. 1, 2017, p. 15. ResearchGate, https://www.researchgate.net/publication/313602417_Corrosion_Protection_Systems_and_Fatigue_Corrosion_in_Offshore_Wind_Structures_Current_Status_and_Future_Perspectives. Accessed 4 Feb. 2025.