Comparative Life Cycle Analysis and Multi-Criteria Decision Analysis of Reinforced Concrete, Steel, and Timber Columns for an Apartment Building in Berlin
Seychelle Ann Dagus | Student Number: 0508078 | s.dagus@campus.tu-berlin.de
1. Introduction
The construction industry significantly impacts the environment, accounting for 30–40% of global energy consumption, 40% of greenhouse gas emissions, and extensive use of natural resources.[1] It is also a major source of waste generation, emphasizing the urgent need for more sustainable methods. Life Cycle Analysis (LCA) is a tool that can help tackle these issues by looking at the environmental impacts of materials and systems throughout their entire lifecycle—from raw material extraction to disposal.
For this project, LCA is used to evaluate three column design options for residential buildings: Reinforced Concrete Columns (RCC), Steel Columns, and Timber Columns. These columns are important structural elements in building design and their environmental impacts depend on the materials and processes used.
LCA is an effective tool, but it also has limitations that can affect the accuracy of analyses. These limitations include the limited availability of region-specific data.[2] However, this study aims to compare LCA and Multi-Criteria Decision Analysis (MCDA) to understand which column designs analyzed are the most sustainable and practical, giving adequate performance over time with the least environmental impact.
The study adopts the LCA methodology, which evaluates the environmental impacts of materials and processes throughout their lifecycle. This method follows the ISO 14040 standards and includes four key phases: defining the goal and scope, creating a lifecycle inventory, assessing lifecycle impacts, and interpreting results.[3]
1.1 Defining the goal and scope
The main goal of this study is to assess and compare the environmental effects focusing on the carbon footprints of three different column design options for use in residential buildings: reinforced concrete columns (RCC), steel columns, and timber (glulam) columns. This comparison will help designers and engineers choose the most practical and environmentally friendly design of columns before the building’s construction starts.
The scope and system boundary is limited to the pre-construction, construction, and operational phases, including raw material extraction, material processing, column fabrication, and operation and maintenance. The end-of-life stages are excluded due to a lack of data and resources. Also, the initial phases have significant environmental impacts and are necessary for identifying sustainable material choices.
1.2 Design Options
The design options for the column chosen to be analyzed are as follows in Figure 3.
According to an LCA study for timber, steel, and reinforced concrete[4], the following specifications are used for the materials and the cross-sectional areas. The columns (18m) were assumed to be pinned to the foundations and the foundations are reinforced concrete isolated footing.
2. Life Cycle Timeline
Establishing interventions and lifespan in LCA is important because it creates the sequential framework and scope for evaluating the environmental and economic impacts of the building. Different materials deteriorate at varying rates based on environmental exposure and usage. To ensure that the analysis represents the real-world performance of a material, it is necessary to consider the maintenance, repairs, and replacement. Having these interventions visually present will also enable us to see the difference in performance between the materials (RCC, Steel, Timber), making it easier to make decisions based on long-term impacts and costs.
2.1 Setting Interventions and Lifespan
2.2 System Lifespan with Interventions in Shiny
3. Life Cycle Inventory and Analysis
After establishing the lifecycle inventory of materials and the interventions throughout the lifespan of the different column materials, the result from the Life Cycle Inventory and Analysis (LCIA) such as Energy, CO2, NOx, and SO2 emissions were obtained.
Figure 5 shows the Energy Consumption and the emissions of the Reinforced Concrete Column (RCC), Steel Column (SC), and Timber Column (TC). The results show noticeable variations in the environmental performance of the three material options.
Energy Consumption: SC shows the highest energy consumption among the three material options, meaning that material extraction, manufacturing, and processing to produce steel require very high energy consumption. RCC consumes less energy than SC but is still significantly higher than TC. This includes cement production which also requires demanding energy. TC shows its natural nature and being a renewable material obtained the lowest energy consumption, showing its advantage as a sustainable alternative for energy efficiency in construction. Therefore, SC having the highest energy consumption makes it less favorable for sustainable construction unless renewable energy is used in production.
CO2 Emissions: When it comes to CO2 emissions, SC is the highest in terms of CO2 emissions, followed by RCC, and TC is the lowest in terms of emissions. The combustion of fossil fuels and the chemical processes used in the production of steel are the causes of SC’s high CO2 emissions. The CO2 from RCC is mainly caused by cement production where CO2 is deposited. On the other hand, TC has a negative value of CO2 because it stores carbon during tree growth. Therefore, in terms of carbon footprint, TC is the most sustainable option, whereas SC is the least desirable.
NOx and SO2 Emissions: It is noticeable that SC has a high difference with RCC and TC in terms of NOx and SO2. For the same reason, the emissions obtained from SC are mainly because of steel manufacturing. RCC has only moderate emissions while TC shows minimal emissions that are mainly related to transportation and light processing of timber. This demonstrates that steel production contributes significantly to air pollution and acid rain. TC’s emission proves that it is the most environmentally friendly option.
4. Multi-Criteria Decision-Making (MDCM) – Analytic Hierarchy Process (AHP)
To evaluate and obtain the best option among the three column design options (RCC, SC, and TC), the Analytic Hierarchy Process (AHP) is utilized. This MCDM method gives a structured framework for comparing alternatives based on multiple performance criteria such as energy consumption, CO2, NOx, and SO2 emissions.
4.1 Structure the Decision Problem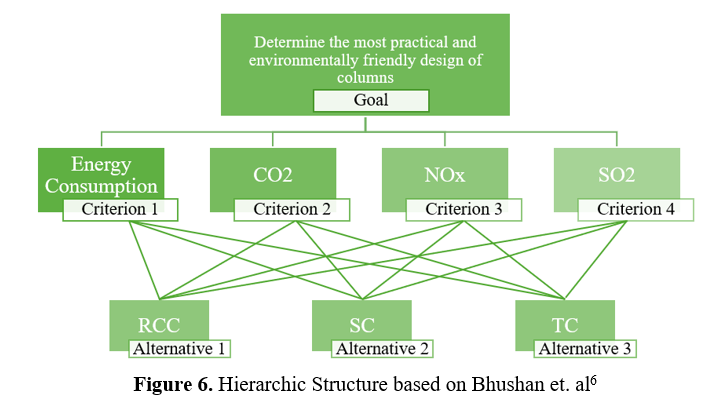
4.2 Pairwise Comparison
Criteria Weights Matrix
The criteria weights matrix captures the relative importance of performance indicators based on project goals and engineering considerations. Using Saaty’s scale, the weights are distributed to quantify the preferences between pairs of criteria.
4.2 AHP Results
Using the AHP method, the following priority weights for the alternatives are obtained:
TC achieves the highest priority due to its low energy consumption, negative CO2 emissions, and minimal NOx and SO2 emissions. This makes TC the most sustainable option for environmentally conscious construction in Berlin.
RCC is not as environmentally friendly as TC, but it balances environmental performance and lifecycle costs. It is a practical choice when the lifecycle cost is the priority in construction.
SC ranks lowest due to its high energy demands and emissions, but it remains necessary for projects requiring high strength and durability.
References:
[1] Ortiz, O., Castells, F., & Sonnemann, G. (2009). Sustainability in the construction industry: A review of recent developments based on LCA. *Construction and Building Materials, 23*(1), 28-39.
[2] Gámez-García, D., Gómez-Soberón, J., Corral Higuera, R., Almaral-Sánchez, J., Gómez-Soberón, M., & Gómez Soberón, L. (2015). LCA as a comparative tool for concrete columns and glulam columns. Journal of Sustainable Architecture and Civil Engineering, 11(2), 21-31.
[3] Klöpffer, W., & Grahl, B. (2014). Life cycle assessment (LCA): A guide to best practice. Wiley-VCH.
[4] Abdelfattah Hegeir, O., Kvande, T., Stamatopoulos, H., & Bohne, R. A. (2022). Comparative life cycle analysis of timber, steel and reinforced concrete portal frames: A theoretical study on a Norwegian industrial building. Buildings, 12(5), 573.
[5] Clean Energy Wire. (2024, January 1). Germany bets on timber to advance decarbonisation in construction sector. Clean Energy Wire. https://www.cleanenergywire.org/news/germany-bets-timber-advance-decarbonisation-construction-sector
[6] Bhushan, N., Rai, K., & Cahyono, S. (2022). 2 The Analytic Hierarchy Process. In Strategic decision making: Applying the analytic hierarchy process (Decision engineering). Springer.
[7] Saaty, R. W. (1987). The analytic hierarchy process—What it is and how it is used. Mathematical Modelling, 9(3–5), 161–176. https://doi.org/10.1016/0270-0255(87)90473-8
| Main Page | Introduction | Integration Context of the Civil Systems | Integrated Maintenance Strategies | Life Cycle Analysis | Multi-Objective Optimization | Engineering Reflections and Recommendation |